“If I have seen further than others, it is by standing upon the shoulders of giants.” – Issac Newton
While in elementary school, I learned of the giants in science. There was Newton himself, along with Galileo, Copernicus, and even Aristotle whose ideas were overturned by the great Renaissance scholars. Yet one name overlooked was James Clerk Maxwell. This is an oddity, as we frequently experimented in class with the concepts of electricity and magnetism. Maxwell’s work, expressed in four equations, provides all the theoretical underpinning for the properties of electromagnetic waves. Much of the technological transformation of everyday life in the 20th century is influenced one way or another by Maxwell’s breakthroughs.

When Maxwell published his A Dynamical Theory of the Electromagnetic Field a few months before the end of the American Civil War, his theory did not take the form of the four equations so familiar to physics students. Maxwell presented his ideas in twenty equations. The simplification occurred a couple decades later as Oliver Heaviside made significant advancements in vector calculus. This allowed Heaviside to simplify, to the good fortune of future physicists, Maxwell’s results.
Maxwell’s work highlighted the key difference between electric and magnetic fields. Electric fields can flow away or towards a point source. An electric field will flow away from a positive charge but flow towards a negative charge. Consequently, if you surround an electric charge with a closed surface, the electric flux (number of field lines) leaving that surface will be proportional to the charge.

Friction can cause a migration of negatively charged electrons from one material to another creating static electricity. This is what happens to cloths in a dryer as the material with an excess of negative charges will cling to the material with an excess of positive charges as opposite charges attract. Cling free sheets release a lubricant to decrease friction in the dryer so there is no buildup of static electricity. Another household item, the refrigerator magnet, generates a different type of field also explained by Maxwell.
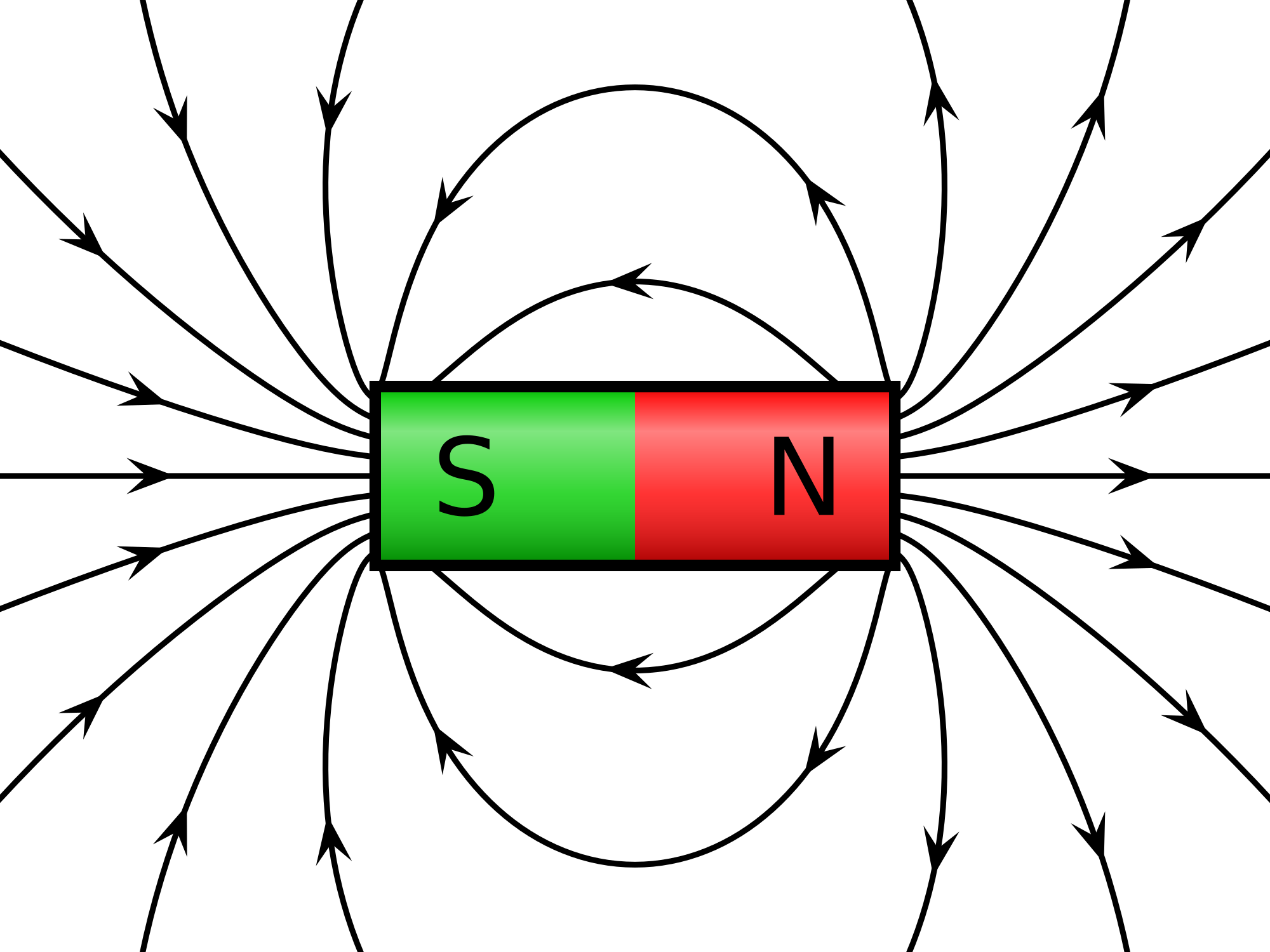
An example of this is the Earth, which acts like a giant bar magnet. However, the Earth’s magnetic field is continually buffeted by the solar wind which deforms its shape. On the day side facing the Sun, the magnetic field is compressed. On the night side facing away from the Sun, the magnetic field is blown away by the solar wind into a tail much like a comet. As the solar wind is constantly changing velocity and direction, the Earth’s magnetic field is dynamic in nature as can be seen below.

Maxwell was able to demonstrate through his equations how a changing magnetic field will produce electric fields. In the case of the Earth, a perturbation of the magnetic field by a strong solar event can induce electric fields in the atmosphere. In 1859, just six years before Maxwell published his work on electromagnetic fields, the strongest magnetic event in recorded history occurred. On September 1st, from his solar observatory 25 miles south of London, Richard Carrington observed a major solar flare. The following night, the disruption in the Earth’s magnetic field produced aurora as far south as Cuba and induced electrical currents to the extent that telegraph operators were able to send messages even when the batteries were disconnected. The original New York Times report on the magnetic storm can be read here.
A more recent event transpired in 1989 when a magnetic storm caused a twelve hour blackout in the province of Quebec and aurora displays as far south as Florida. The disturbance in the Earth’s magnetic field induced an overload in the electrical grid creating the blackout. As society becomes more and more dependent on electrical devices such as cell phones, space weather forecaster takes on a greater significance. A repeat of the 1859 event would cause significant disruption in the electrical grid and is why a premium is placed on producing better space weather prediction models.
While a changing magnetic field will produce an electric field, a changing electric field also produces a magnetic field. When combined, changing electric and magnetic fields form something of a symbiotic relationship which produces an electromagnetic wave. An example of such is below.

The term electromagnetic (EM) wave may sound foreboding, but we experience these waves in our daily lives in the form of radio, microwave, light, ultraviolet, and x-rays. What differentiates between one type of wave and another is the wavelength. Long wavelength radiation, such as radio, are low in energy while short wavelength radiation, such as x-rays, are high in energy.

During Maxwell’s life, light (visible, infrared, and ultraviolet) was the only EM radiation known to exist. The hallmark of a valid theory is to make successful predictions and in Maxwell’s case, his theory predicted other forms of electromagnetic radiation that would have the same properties as light. That it could be diffracted, reflected, polarized, and would have the same velocity. Heinrich Hertz discovered radio and microwaves in 1888, Wilhelm Roentgen discovered x-rays in 1895, and Paul Villard discovered gamma rays in 1900. Astronomers, no longer restricted to observing the universe in the optical range of the EM spectrum, now use an armada of radio, infrared, ultraviolet, and x-ray telescopes to obtain a much deeper understanding of the heavens. The video below demonstrates how the Crab Nebula appears in various parts of the EM spectrum.
Another prediction from Maxwell’s work would spark a revolution in physics in the 20th century. His equations calculated the speed of light (or any other EM wave) to be a constant at 3.00 × 108 m/s (186,282 miles per second). The key here is the word constant, which means the speed of light is the same regardless of your velocity towards or away from it. To use an analogy, if you are driving on a highway at a speed of 60 mph, and the car in the next lane is traveling at 65 mph, you will be passed by that vehicle at a rate of 5 mph. Now, lets pretend you are traveling in a car exactly 5 mph less than the speed of light. If a light beam was next to you, your life experience would make you think the light, just like the car, would pass you at a rate of 5 mph. However, your intuition would be wrong, the light beam would pass you at the speed of light just the same as if you were standing still.
What gives?
This was a mystery that baffled physicists for half a century and one reason it took a few decades for Maxwell’s work to be fully accepted. Maxwell predicted EM radiation could travel in a vacuum at the speed of light. The prevailing wisdom at the time was EM radiation would need a medium, such as air provides for a sound wave, and it would travel at different velocities relative to its direction in the medium then referred to as ether. In 1887, Albert Michelson and Edward Morley performed an experiment that would measure the speed of light throughout the year as the Earth orbited the Sun. The concept was to make measurements while the Earth was moving in various directions. The speed of light was expected to be faster as the Earth moved in a direction opposite the ether was moving in. The experiment detected no change in the velocity of light and thus, no medium for light to travel in.
This mystery was solved by Albert Einstein’s theory of special relativity in 1905. Einstein’s theory eschews common intuition and treats the speed of light as a constant and time, along with mass and length, as a variable. So what happens on the highway in Einstein’s world? As you near the speed of light, your clock slows down relative to a bystander next to the highway. So, instead of measuring different velocities for the light beam (the bystander measuring the normal speed of light where you measure it at 5 mph), the light beam travels at the same velocity relative to each person. If you are in the car, your clock will run slower and you will age at a slower rate than the bystander. Confusing? Join the club. We do not experience relativistic effects in our daily lives so our intuition fails us mightily here. And that was the nature of Einstein’s genius to break through that barrier.
Einstein would pay tribute to James Clerk Maxwell by stating his work was, “the most profound and the most fruitful that physics has experienced since the time of Newton”. Einstein would further enhance Maxwell’s reputation by describing his contributions towards lying the foundations of special relativity in his highly accessible 1940 Science article Considerations Concerning the Fundaments of Theoretical Physics.
While figuring out the theory of electromagnetism, Maxwell found the time to make two other key contributions to science. James Clerk Maxwell was the first person to develop a method of taking a color photograph. Maxwell produced a theory that a color photograph could be developed by taking an image with blue, green, and red filters and combining the three to create a single color image. Maxwell demonstrated this technique in a 1861 lecture at the Royal Institution and the result was this.
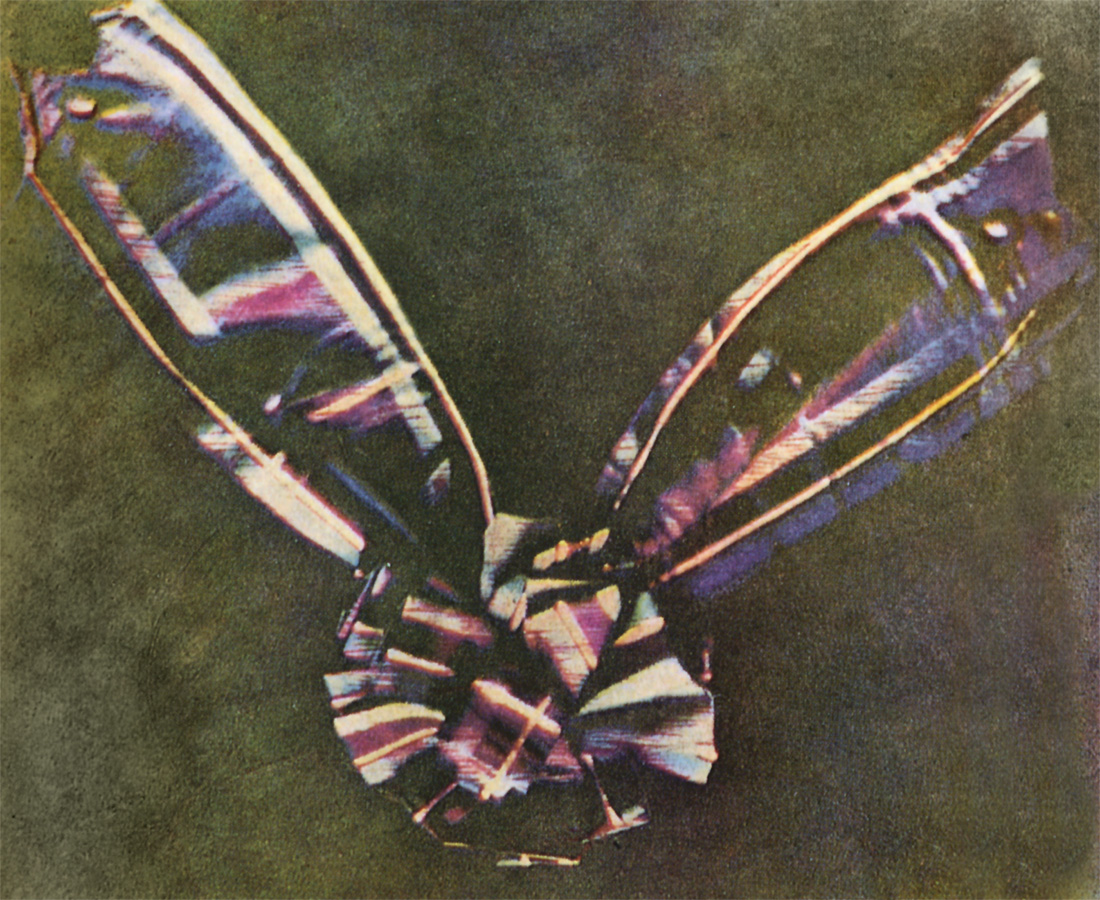
The RGB color scheme was used to develop color television and is still used to produce color space images including the Cassini mission which explored Saturn, its moons, and rings from 2004 to 2017.
During the mid 1800’s, astronomers generally thought that Saturn’s rings were either a solid or a fluid (gaseous). In 1859, Maxwell postulated that the rings were composed of many small, orbiting bodies. Maxwell determined that the forces imposed on a solid ring system around Saturn would be so great as to break apart the rings. Also, Maxwell argued that a ring system of many small, orbiting bodies could give the optical illusion of acting as a fluid. If the rings were one solid piece, the entire ring system would revolve around Saturn at the same rate much like a vinyl record does. A system of small, orbiting bodies would obey Kepler’s laws. This means the inner bodies would orbit Saturn at a faster rate than those located in the outer rings. Maxwell’s theory was proven correct in 1895 when James Keeler’s spectroscopic study of the rings indicated they obeyed Kepler’s Law and thus, were composed of billions of small bodies.

When the Cassini mission took a color image of Saturn’s rings, it validated Maxwell’s theory of the rings, used Maxwell’s technique to produce a color image, and sent the image back to Earth via radio transmissions whose properties are explained by Maxwell’s equations. Maxwell’s ability to unify the forces of electricity and magnetism inspired future physicists to endeavor to unify the other forces of nature, otherwise known as the theory of everything.
Applications of Maxwell’s work is not restricted to physics and astronomy. Any technology using electricity or magnetism are underpinned by Maxwell’s four equations. While Einstein stood on Maxwell’s giant shoulders to produce the theory of special relativity, we stand on Maxwell’s giant shoulders everyday when we turn on a light, a television, or a computer. No doubt, James Clerk Maxwell’s legacy is among the greatest in science.
*Image on top of post is the spiral galaxy M101 formed from a composite of observations from the Hubble Space Telescope (light), Spitzer Space Telescope (infrared), and Chandra X-ray observatories. All these electromagnetic waves follow the properties of Maxwell’s equations. The red is infrared and maps galactic dust. The yellow is light and consists of stars, and blue are x-rays and represent regions of high temperatures such as supernova remnants and black hole accretion disks. This composite provides a complete map of the galaxy. Credit: NASA, ESA, CXC, SSC, and STSci.